One of the hottest topics in the philosophy of chemistry is the relationship between chemistry and physics, in particular, between molecular chemistry and quantum mechanics. The advent of quantum mechanics during the first half of the twentieth century gave rise to the idea that the new theory would account for all the phenomena usually addressed by means of chemical sciences: “The underlying physical laws necessary for the mathematical theory of a large part of physics and the whole of chemistry [are] completely known from quantum mechanics” (Dirac, 1929: 714).
This idea rests on a prominent reductionist assumption according to which chemical entities, when properly analyzed, are nothing beyond quantum physical entities. However, that initial optimism gradually vanished through time: at present, it is broadly known that there are some significant obstacles to explaining chemical facts solely with the tools offered by quantum mechanics. Proponents of a reductionist view might assert those explanation hurdles to be a purely technical matter. Yet, this seemingly uncontroversial view faces serious difficulties once the nature of the involved entities is carefully considered.
According to molecular chemistry, molecules are objects composed of more elementary objects: atoms which are bonded in specific ways. Molecules have a definite structure, manifested by its shape in the three-dimensional physical space. In this scientific context, any molecular structure is defined in part by the spatial location of the constituent atoms relative to one another and the location of the chemical bonds linking those atoms (bond lengths, bond angles, etc.). This structure is strongly related to the corresponding chemical reactivity and the grouping of macroscopic substances as they appear in the laboratory. The molecular geometry is usually represented by colorful ball-and-stick models which, although toy-models, express the geometrical arrangement of the molecule’s components.
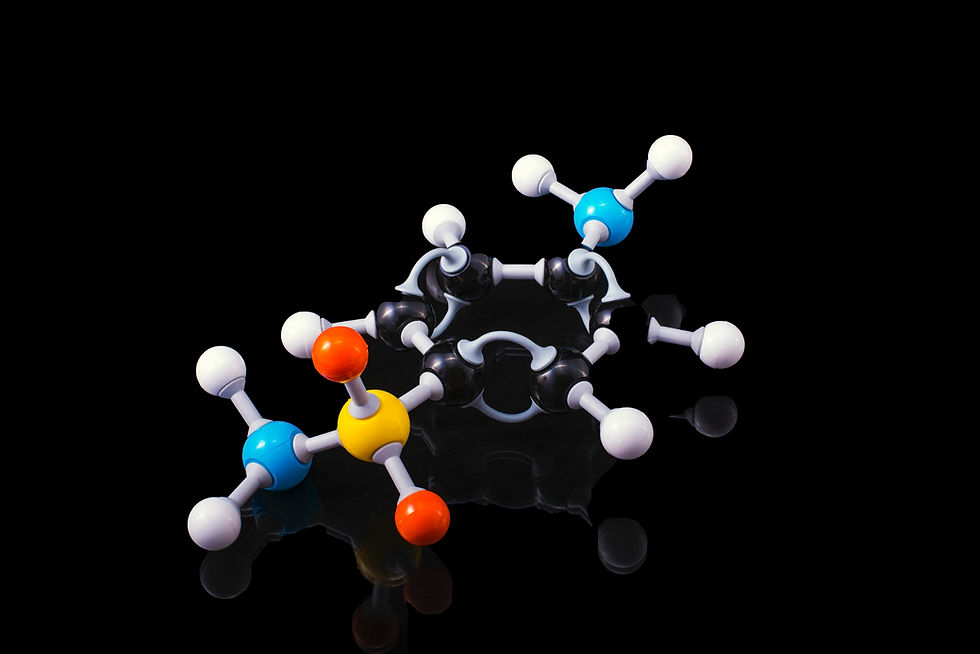
The world of standard quantum mechanics is completely different. Quantum systems cannot be represented in the physical space: for a quantum entity, the Heisenberg indetermination principle states that the simultaneous assignment of a definite position and a definite velocity is logically forbidden by the very structure of the theory. Furthermore, the Kochen-Specker theorem proves the contextuality of quantum mechanics: any assignment of definite values to all the properties of a quantum system leads to contradiction. This is completely inconceivable in the classical world: we cannot imagine, say, a ball with the property of color but without any of the possible colors. In other words, a quantum “particle” is not an individual in the traditional sense, since it has properties with no definite value; and this is not a mere epistemic limitation, but an ontological fact described by the theory.
In the classical world, objects interact with each other; but once the interaction ends, each one acquires an independent behavior. Quantum entities, by contrast, remain entangled after the interaction has ended: their behavior stays correlated despite the fact that there is no longer a physical link between them. The strength of entanglement, unlike physical interactions, does not decrease with the distance between the correlated objects. It is in this sense that the quantum realm is usually said to be holistic: when entangled, quantum systems seem to constitute a single composite whole where the original components have lost their identity.
Classical objects belong to the category of “individual”: there is some principle of individuality that makes them different from others at a certain time and identical to themselves for a long time. This is not an epistemic matter, but an ontological fact that is manifested in the way that classical objects statistically behave: when two classical objects are exchanged, a different situation is obtained. Quantum items, by contrast, may be completely indistinguishable: they cannot be distinguished from others of the same kind and cannot be re-identified through time. In fact, two indistinguishable quantum entities cannot be exchanged as to lead to a different situation; in a sense, one cannot even say that two indistinguishable quantum entities have been exchanged. Furthermore, one cannot say that a given quantum particle detected now is the same as that observed a while before.
Contextuality, entanglement, holism, indistinguishability: these are quantum features completely alien to the world of chemistry. But, what about the appeal to quantum mechanics in chemistry? What about the many efforts to solve the Schrödinger equation in the description of molecules? The fact that some tools coming from quantum mechanics are extensively used in the description of molecules does not make them legitimate quantum items: a molecule is still a system composed by nuclei surrounded by inert inner-shell electrons, placed in definite positions in three-dimensional physical space, and bonds explained in terms of interacting “bonding” electrons; the molecular structure is defined by the spatial arrangement of these elements. So, although this is the notion of molecular structure in the field of chemistry that is closer to QM, it is still a quasi-classical notion: nuclei-with-inner-shell-electrons are treated as distinguishable and non-contextual classical objects, and bonding electrons are conceived as quasi-classical particles. This means that, although the behavior of electrons is governed by the Schrödinger equation, they are placed in physical space and retain their identity in the whole system, with no entanglement among them and neither with respect to the classical nuclei-with-inner-shell-electrons. In other words, despite the role played by quantum notions, the ontological breakdown remains.
Of course, this does not make the idea of reduction unsustainable. The insistent reductionist still has two possible strategies. One is to expect the deep revision of chemistry, which in the future would dispense with any concept not perfectly translatable to the underlying quantum theory; in this case, the chemical concept of molecule will be substituted by a completely quantum notion (Bader 1990). The other is to advocate for the replacement of quantum mechanics in its traditional version with another theory better suited to intertheory links. One candidate for this strategy is Bohmian mechanics (Goldstein 2017), since it describes a world much closer to the classical ontology; however, this implies to take charge of the widely discussed difficulties of this alternative theory.

On the other hand, for those without a particularly strong reductionist temperament, the ontological breakdown between molecular chemistry and quantum mechanics serves to support a non-reductionist view. In this line there are also different options. For instance, one may consider that the non-reducible chemical concepts, such as molecular structure, denote emergent items that, although ontologically depending on the underlying physical realm, populate the world of chemistry which is different from the world of quantum mechanics (Hendry 2006, 2010). Or one may take an ontological pluralist position, according to which chemical concepts refer to items whose existence is guaranteed by the success of chemistry itself and, therefore, do not need to refer to a fundamental physical domain (Lombardi and Labarca 2005, Lombardi 2014).
In summary, many different perspectives regarding the relationship between molecular chemistry and quantum mechanics remain open. What cannot be ignored is the deep ontological breakdown between the worlds of molecular chemistry and of quantum mechanics.
References
Bader, R. F. W. (1990). Atoms in Molecules: A Quantum Theory. Oxford: Oxford University Press.
Goldstein, S. (2017). “Bohmian Mechanics.” In Edward N. Zalta (ed.), The Stanford Encyclopedia of Philosophy (Summer 2017 Edition), URL = <https://plato.stanford.edu/archives/sum2017/entries/qm-bohm/>.
Hendry, R. F. (2006). “Is there downward causation in chemistry?.” In D. Baird, L. McIntyre and E. Scerri (eds.), Philosophy of Chemistry: Synthesis of a New Discipline. Berlin: Springer.
Hendry, R. F. (2010). “Ontological reduction and molecular structure.” Studies in History and Philosophy of Modern Physics, 41: 183-191.
Lombardi, O. (2014). “The ontological autonomy of the chemical world: facing the criticisms.” In E. Scerri and L. McIntyre (eds.), Philosophy of Chemistry: Growth of a New Discipline (Boston Studies in the Philosophy and History of Science). Springer: Dordrecht.
Lombardi, O. and Labarca, M. (2005). “The ontological autonomy of the chemical world.” Foundations of Chemistry, 7: 125-148.
Martínez González, J. C., Fortin, S., and Lombardi, O. (2019). “Why molecular structure cannot be strictly reduced to quantum mechanics.” Foundations of Chemistry, 21: 31-45.
* This article is inspired by our published paper “Why molecular structure cannot be strictly reduced to quantum mechanics” (see References).
Juan Camilo is Assistant Researcher of the National Council of Scientific and Technical Research, Argentina.
Olimpia is Superior Researcher of the National Council of Scientific and Technical Research, Argentina.
Comments